RESEARCH
RESEARCH STATEMENT
As global warming intensifies1,2 and cooling demand increases,3 the need for cost-effective, zero global warming potential (GWP) air conditioning systems is dire. A recent study showed that to curb air conditioning emissions, these systems should eliminate the use of high GWP refrigerants, utilize more efficient methods of cooling, and implement more efficient methods of dehumidification.4 Unfortunately, while new hydrofluoroolefin refrigerants have lower GWPs, they degrade into problematic chemicals, like trifluoroacetic acid.5 Meanwhile, researchers have been investigating new refrigeration cycles6,7 to provide efficient, low-GWP refrigeration, but few of these new cycles also provide efficient dehumidification. During my Ph.D., I invented, theoretically analyzed, and experimentally demonstrated a new air conditioning cycle that efficiently provides both cooling and
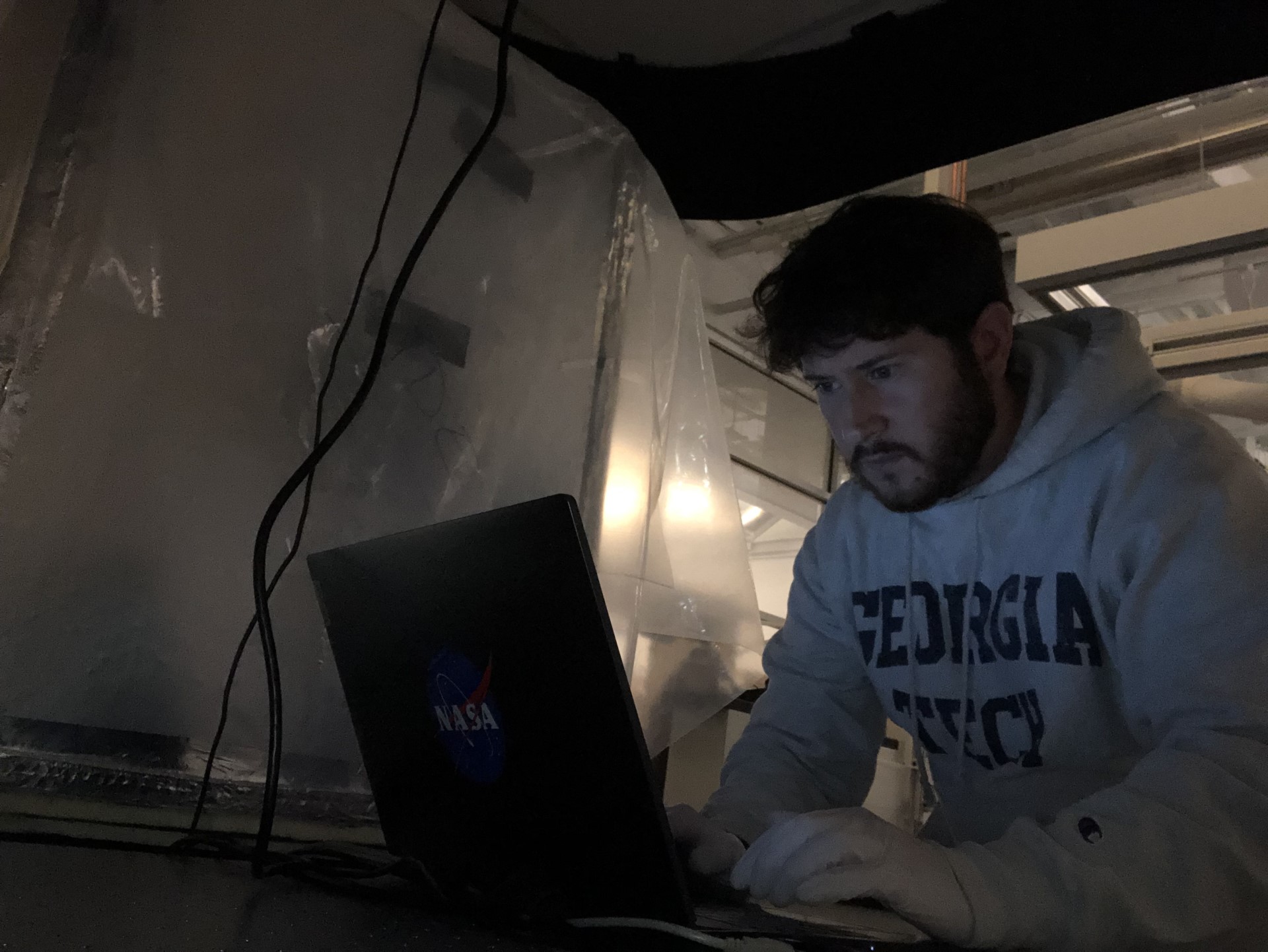
dehumidification, uses zero GWP refrigerants, and is powered by low-grade solar heat. I’ve also explored the integration of thermal storage into air conditioning applications and the materials that will drive down costs, as well as a modification to my air conditioning cycle that allows it to be used for desalination or atmospheric water harvesting. With my expertise in thermodynamics, heat and mass transfer, and technoeconomics, I am uniquely positioned to investigate groundbreaking technologies that span the entire water-energy nexus.
Dissertation Work: Refrigeration and Dehumidification Using Thermal Phase Separation
Mixtures that possess a lower critical solution temperature (LCST) are homogeneous at lower temperatures but phase separate when heated. This enables a new air conditioning cycle that uses LCST mixtures that act as a desiccant at ambient temperatures, absorbing moisture and dehumidifying air. Then, when heated above the LCST, the mixtures separate, giving a nearly pure water phase that can be evaporated into outdoor air. This both rejects the moisture absorbed during dehumidification and produces refrigeration through an indirect evaporative cooling effect. I began my Ph.D. by investigating the fundamental thermodynamics of this new cycle, and then I used these results to propose the idea to the DOE and won the IBUILD Fellowship to demonstrate this cycle. I built a proof-of-concept LCST cycle air conditioner that produced simultaneous refrigeration and dehumidification. I published the findings (from thermodynamics to experimental results) in various publications. The thermodynamic analysis reveals that we need new LCST mixtures to improve performance, enabling lower indoor temperatures and humidities than what current mixtures can achieve. These new mixtures need mixing enthalpies and mixing entropies of greater magnitude than existing LCST mixtures, which I intend to continue exploring.
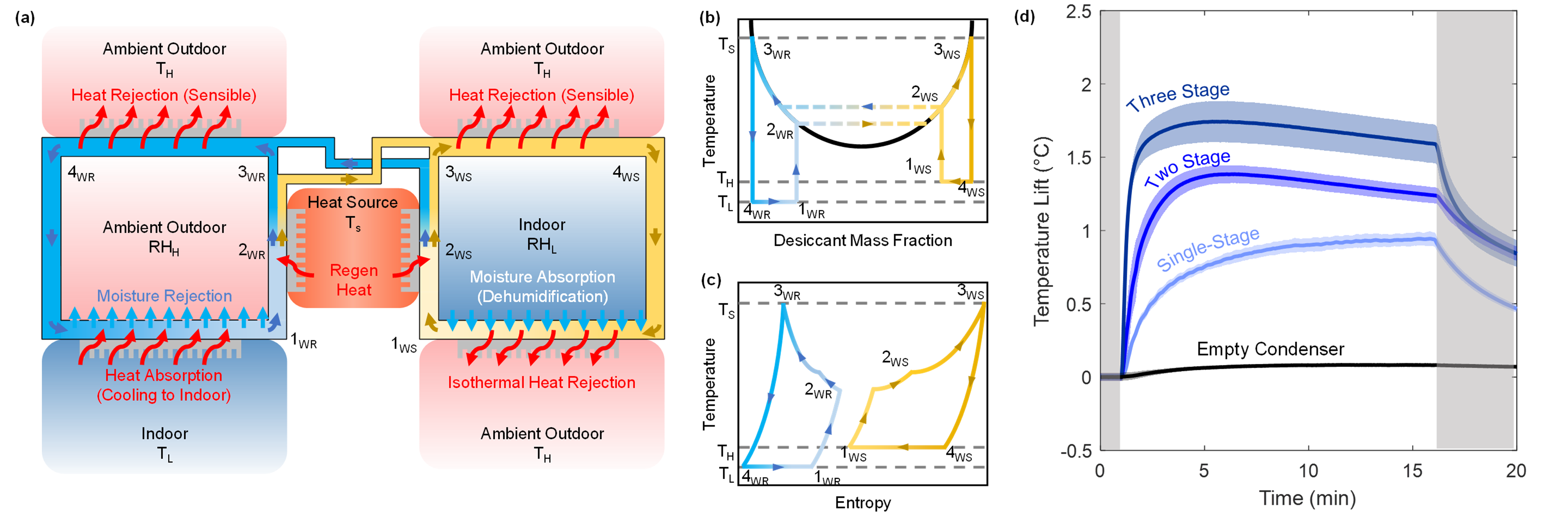
Fig. 1. LCST air conditioning cycle. (a) Schematic. (b) Phase diagram. (c) T-S diagram. (d) Experimental results.
Other Projects:
Thermal Energy Storage
Buildings accounting for 39% of primary energy in the U.S., and 50% of building energy is utilized in thermal end uses. Thermal energy storage can provide both cost and energy savings by shifting thermal loads to periods when systems are more efficient and energy is cheaper. However, the cost of thermal storage needs to drop before it can become an attractive option. While at Georgia Tech, I led an effort to quantify the different contributions to the system cost of a thermal battery (a collaboration with NREL). While many researchers have been focusing on optimizing thermal storage materials (an important effort, no doubt), this analysis provides a more wholistic approach. We found that the cost of heat exchangers tend to dominate; in this case, a cheaper storage material will do little to decrease the system cost. We developed a regime map and determined the group of storage material properties that when increased would lower system cost (figure-of-merit), based on which system component is the dominating cost. When heat exchangers dominate cost, it is actually the thermal effusivity that should be maximized, rather than the storage capacity per unit cost of the storage material.
Desalination
With water stress increasing around the globe,8 it becomes increasingly important that we secure new, cost-effective methods of artificially producing fresh water to complement our natural resources. Desalination has proved to be one of the most cost-effective methods of producing fresh water (with heavy adoption in Gulf countries9 that have little access to natural fresh water), owing largely to developments in reverse osmosis systems and the decrease in energy consumption that
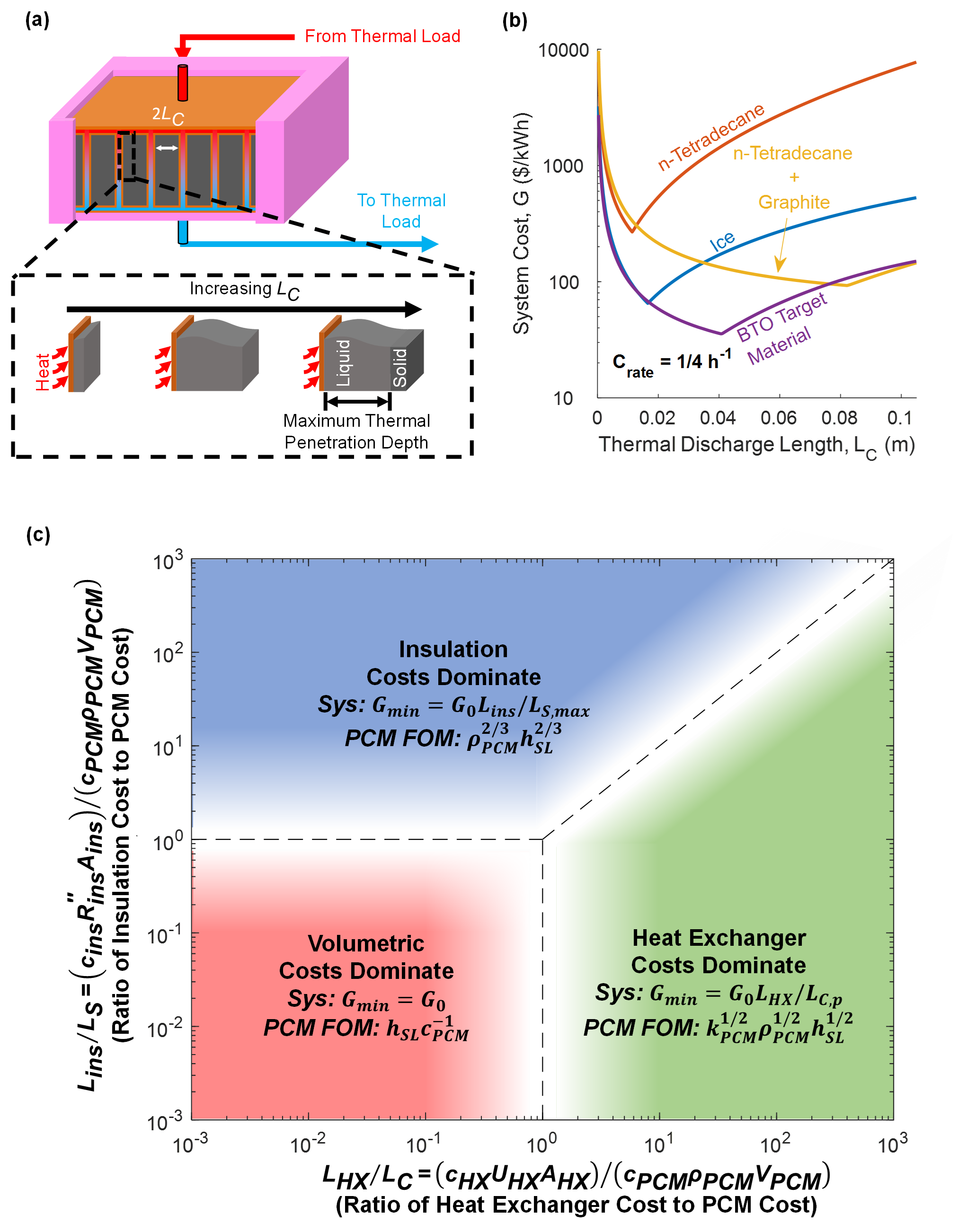
Fig. 2. (a) Thermal penetration depth in a thermal battery. (b) Cost of a thermal battery with various storage materials as a function of thermal discharge characteristic length (PCM thickness). (c) Thermal battery cost regime map.
has accompanied these innovations. However, as we begin desalinating more inland, brackish water sources, brine disposal becomes an issue. Zero liquid discharge, the method of separating almost all of the water from the salt and then disposing of the solid salt byproduct, has been shown to be one of the most cost-effective options for inland desalination brine management.10 Currently, reverse osmosis membranes are unable to achieve zero liquid discharge, due to the high pressure required.
Thermal desalination processes, while somewhat more expensive at treating seawater than reverse osmosis, have proven to be effective methods of brine concentration, which is necessary to achieve zero liquid discharge. During my Ph.D., I have studied several thermal desalination brine concentration systems. First, I helped model an air gap diffusion distillation system and highlight the benefits when compared to its membrane counterpart. Next, I worked on using LCST mixtures for desalination. One important finding was my discovery that adding salt to an LCST mixture decreases the chemical potential of both phases after separation. While this discovery came while researching my LCST air conditioning cycle, I also realized its potential benefits for desalination and devised a multi-stage forward osmosis desalination system using LCST mixtures that could achieve zero liquid discharge.
Atmospheric Water Harvesting
Because of my interest in desalination and dehumidification, I naturally gravitated towards atmospheric water harvesting, which is the process of converting water vapor (humidity) to clean, drinkable water. In a recent perspective, I show that atmospheric water harvesting faces an uphill battle if it is to compete with desalination, based on the fundamental thermodynamic limitations and corresponding economics (Fig. 1).11 However, I did find that atmospheric water harvesting can fill certain niches, and systems that utilize cheaper heat sources and have higher exergetic efficiencies could theoretically compete with desalination. To this end, I have shown how my LCST dehumidification cycle can also be used for atmospheric water harvesting,12 with high exergetic efficiency (owing to the thermally induced phase separation) and low-cost solar heat.
Exergy
During my Ph.D., I came to appreciate how powerful a tool exergy is for researchers studying thermodynamic systems. I have utilized it to analyze various systems, from my LCST refrigeration and dehumidification cycle to atmospheric water harvesting and desalination. Unfortunately, exergy can be difficult to conceptualize for many students and is often a mere afterthought in (or excluded completely from) undergraduate
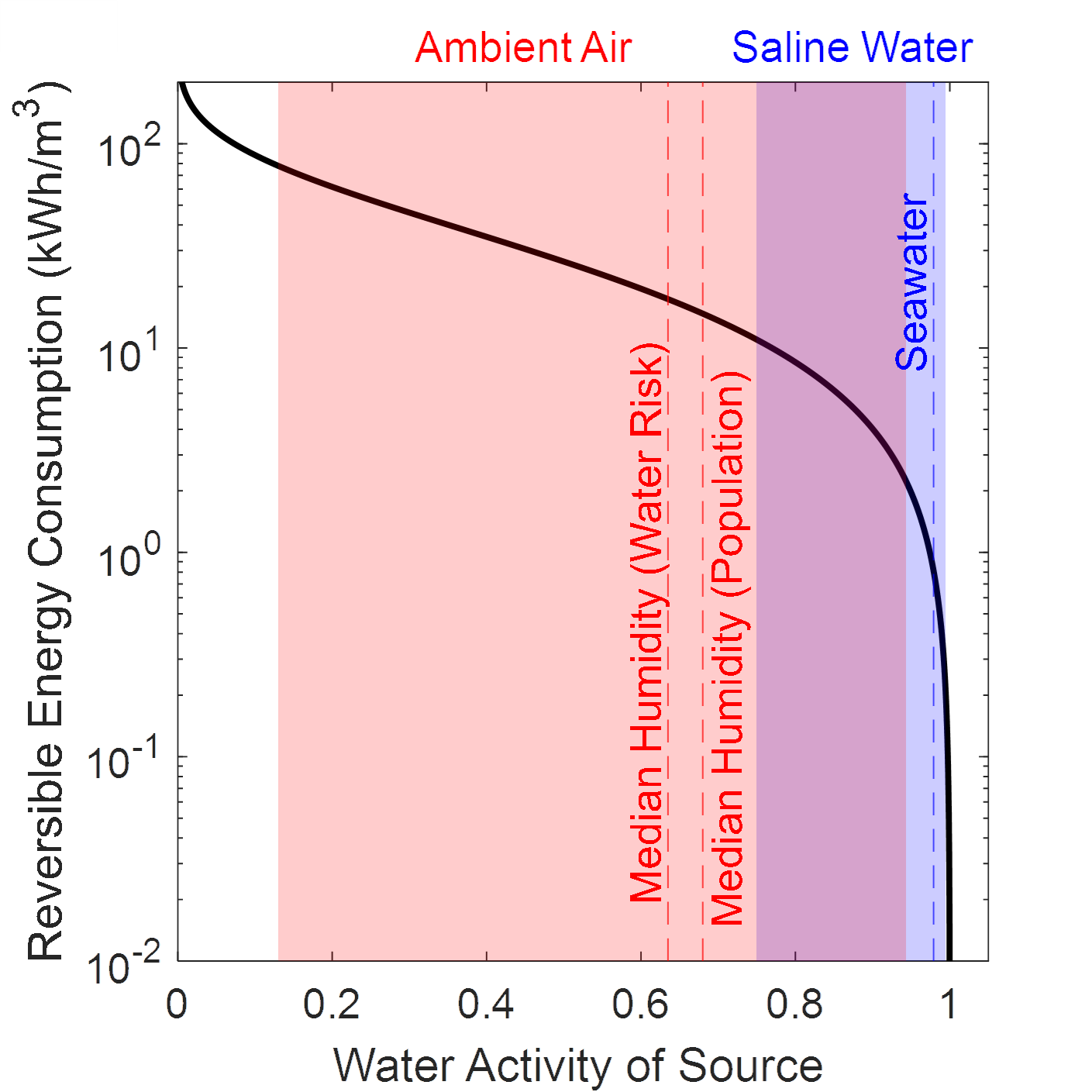
Fig. 3. Reversible energy consumption of water separation, with the activities associated with desalination and atmospheric water harvesting highlighted in blue and red, respectively.
thermodynamics courses. Tu and Chen recently provided a critique of exergy and proposed a new quantity called “useful energy loss”. I wrote a commentary on their analysis, demonstrated that a proper exergy accounting will produce the same value as their useful energy loss term, and in so doing, I discovered an analogy between exergy flow in thermodynamic systems and the flow of current in electrical circuits. I believe this new method of conceptualizing exergy has the potential to cut across both research and teaching, providing new methods of visualizing and solving exergy flow in thermodynamic cycles.
Proposed Research: My proposed research includes various projects within the water-energy nexus. First, I aim to develop new technologies to reduce the emissions of thermal energy use in the built environment. This includes novel refrigeration, dehumidification, and heat pump technologies, such as the LCST cycle that I invented during my Ph.D., to provide inexpensive, emission-free cooling and heating. This also includes thermal energy storage, which can shift thermal loads to periods of higher efficiency, with lower costs than existing thermal storage systems. My second research thrust will involve new technologies for producing fresh water. This will include both desalination and atmospheric water harvesting technologies, some of which will be powered by the thermally-induced phase separation I studied during my Ph.D.
Separation-Driven Refrigeration
I plan to continue my research on separation-driven refrigeration cycles in pursuit of an air conditioning cycle with ultra-low emissions (zero GWP refrigerants, high COP). This will include continued research on my LCST cycle by investigating new LCST mixtures that can achieve lower indoor temperatures and humidities. I anticipate this effort will be a collaboration with my colleagues at Georgia Tech who are experts in Molecular Dynamics simulations of ionic liquids (a collaboration that is already underway). As better mixtures are discovered, I will utilize my prior knowledge to develop a practical, cost-effective LCST air conditioning system.
I also propose to investigate different separation methods for new refrigeration cycles. Solar heating is not cost-effective in all locations, whereas electrically-driven separation technologies have proven to be very cost-effective and energy efficient. These technologies could prove critical in the quest to find sustainable refrigeration and dehumidification cycles. My lab will investigate work-driven dehumidification, such as mechanical vapor compression regenerated desiccants and membrane dehumidifiers.
Water
As mentioned above, I plan to continue researching new LCST mixtures. Aside from their benefits in the air conditioning cycle, they would also enable new LCST desalination and atmospheric water harvesting technologies. By investigating novel water production systems that utilize LCST mixtures, my lab will work towards the creation of new technologies that can lower the cost of water while also decarbonizing water production (since LCST systems can be powered by solar heat). I also intend to continue my research on more traditional desalination technologies for brine management, such as air gap diffusion and membrane distillation.
Paradigm-Shifting Thermal Energy Storage
My prior cost-scaling analysis of thermal storage revealed that (i) heat exchangers tend to dominate cost, in which case (ii) a more energy dense, thermally conductive material would decrease the system cost. Thermochemical storage materials have energy densities that dwarf sensible and phase change storage materials, presenting the opportunity to create a thermal battery in which heat exchanger costs are no longer the limit factor. I intend to leverage the experience I gained with thermochemical storage materials during my Ph.D. to develop cost-effective thermal batteries.
Additionally, the separation-driven refrigeration cycles that I invented have the potential to have built-in thermal storage capacity. By separating the two phases during periods of excess energy, high efficiency operation, and/or low energy costs, the two phases can be stored (acting as thermochemical storage materials) with zero self-discharge. In this case, the thermal storage is directly integrated with the evaporator and condenser of the system, eliminating the heat exchanger cost that dominates other thermal storage systems.
Collaborations and Funding Sources: My research lies at the intersection of several disciplines, including Mechanical Engineering, Materials Science, Polymer Physics, and Economics, spanning several institutions, from universities to national laboratories. My research has greatly benefited from these collaborations, and I intend to continue these collaborations and form new ones when forming my own research group. Through my IBUILD Fellowship, I have had the opportunity to collaborate with researchers at the National Renewable Energy Laboratory (NREL), visit Oak Ridge National Laboratory (ORNL) to present my research, and participate in the Department of Energy (DOE) Building Technologies Office (BTO) Peer Review conference, at which I presented my work and interfaced with researchers working on topics that complement my own. I plan to continue collaborating with NREL, utilize my status as an IBUILD fellow to form new collaborations with researchers at ORNL, and collaborate with other IBUILD fellows (who have now gone into academia, national labs, and industry). Additionally, I have collaborated with researchers at Georgia Tech (Mech. Eng., Chemistry, Mat. Sci.), Arizona State University (Mech. Eng., Chem. Eng.), University of Virginia (Mech. Eng.), UC Berkeley and Lawrence Berkeley National Laboratory (Chemistry) and have identified the potential for new collaborations with groups at University of South Alabama (Chem. Eng.) and Colorado School of Mines (Mech. Eng.).
I anticipate that the majority of my funding will come from federal government grants, mainly from DOE. Throughout my Ph.D., I have worked with my advisors to write grant applications from DOE BTO and ARPA-E. Much of my research (from cooling to thermal storage) involves building technologies, making DOE BTO a prime target for funding. Much of my research also involves new, groundbreaking thermodynamic cycles, making ARPA-E and DOE Basic Energy Sciences great sources of funding as well. I intend to utilize the connections I have made within the DOE as an IBUILD fellow to aid me in securing this funding. I also intend to target NSF grants for smaller-scale projects and research topics that fall outside of DOE’s funding scope. For projects dealing with clean water production (desalination and atmospheric water harvesting), I intend to also target private industry, philanthropic organizations, and the DOD, since all of these organizations have an interest in reliable, cost-effective technologies for producing fresh water. Finally, I want to encourage my students to apply for various graduate research fellowships (NSF GRFP, DOE IBUILD, DOD NDSEG). By leaning on my experience successfully winning one of these fellowships, I am confident that I will be able to help my students win them as well.
References:
(1) Hansen, J.; Sato, M.; Ruedy, R.; Lo, K.; Lea, D. W.; Medina-Elizade, M. Global Temperature Change. Proc. Natl. Acad. Sci. 2006, 103 (39), 14288–14293. https://doi.org/10.1073/pnas.0606291103.
(2) IPCC. Climate Change 2014: Synthesis Report, 2014.
(3) The Future of Cooling – Analysis. IEA. https://www.iea.org/reports/the-future-of-cooling (accessed 2022-07-26).
(4) Woods, J.; James, N.; Kozubal, E.; Bonnema, E.; Brief, K.; Voeller, L.; Rivest, J. Humidity’s Impact on Greenhouse Gas Emissions from Air Conditioning. Joule 2022, 6 (4), 726–741. https://doi.org/10.1016/j.joule.2022.02.013.
(5) Eisemann, L. Persistent degradation products of halogenated refrigerants and blowing agents in the environment: type, environmental concentrations, and fate with particular regard to new halogenated substitutes with low global warming potential; Umweltbundesamt, 2021.
(6) Rajan, A.; McKay, I. S.; Yee, S. K. Continuous Electrochemical Refrigeration Based on the Brayton Cycle. Nat. Energy 2022, 7 (4), 320–328. https://doi.org/10.1038/s41560-021-00975-7.
(7) Lilley, D.; Prasher, R. Ionocaloric Refrigeration Cycle. Science 2022, 378 (6626), 1344–1348. https://doi.org/10.1126/science.ade1696.
(8) Water Scarcity. UN-Water. https://www.unwater.org/water-facts/water-scarcity (accessed 2023-02-09).
(9) DC, A. C. W. The Costs and Benefits of Water Desalination in the Gulf. Arab Center Washington DC. https://arabcenterdc.org/resource/the-costs-and-benefits-of-water-desalination-in-the-gulf/ (accessed 2023-08-03).
(10) Menon, A. K.; Jia, M.; Kaur, S.; Dames, C.; Prasher, R. S. Distributed Desalination Using Solar Energy: A Technoeconomic Framework to Decarbonize Nontraditional Water Treatment. iScience 2023, 26 (2), 105966. https://doi.org/10.1016/j.isci.2023.105966.
(11) Kocher, J. D.; Menon, A. K. Addressing Global Water Stress Using Desalination and Atmospheric Water Harvesting: A Thermodynamic and Technoeconomic Perspective. Energy Environ. Sci. 2023, 16 (11), 4983–4993. https://doi.org/10.1039/D3EE02916F.
(12) Kocher, J. D.; Yee, S. K.; Wang, R. Y. A First and Second Law Analysis of a Thermoresponsive Polymer Desiccant Dehumidification and Cooling Cycle. Energy Convers. Manag. 2022, 253, 115158. https://doi.org/10.1016/j.enconman.2021.115158.
Research funded by the Innovation in Buildings Graduate Research Fellowship
The IBUILD Fellowship is made possible by:
- DOE-Building Technology Office (BTO)
- Oak Ridge Institute for Science and Education (ORISE)
- Oak Ridge Associated Universities (ORAU)